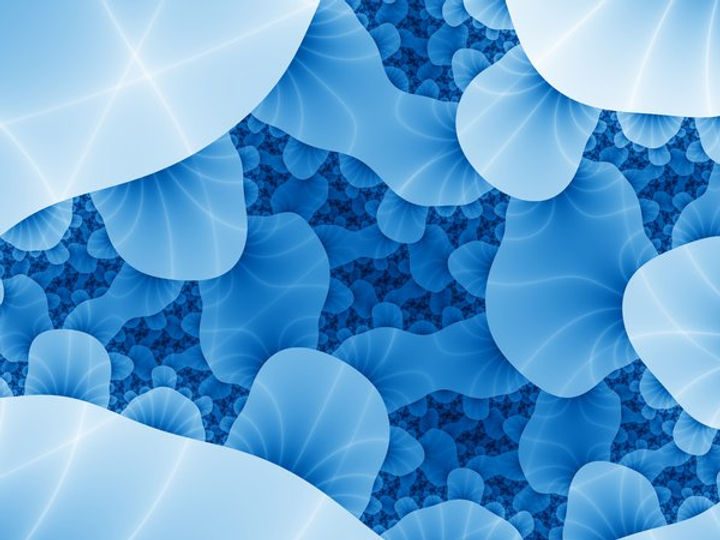
IMMUNOFLUORESCENCE TECHNIQUE
Fluorescence and phosphorescence are both types of luminescence. When molecules with luminescent properties absorb light, they emit light of a different wavelength. With fluorescence the emission of light occurs extremely rapidly after the absorption of excitation light, whereas with phosphorescence emission continues for milliseconds to minutes after the energy source has been removed. Fluorescent materials give off light because of their atomic structure. Electrons are arranged in discrete energy levels surrounding the atom’s nucleus with each level having a predetermined amount of energy. When an electron absorbs the energy from a photon of light (Figure 1) it
becomes “excited” and jumps to a higher, less stable energy level.
The excited state does not last long. The half-life of the excited state is generally less than 10 seconds. The electron loses a small amount of energy as heat and the remainder of the extra energy is given off in the form of a photon. The emitted fluorescence has a lower energy than the absorbed light, so the wavelength of the emitted light is longer than that of the excitation light (except in the case of multi-photon excitation).
Figure 1. Principle of fluorescence.
A range of wavelengths of light can excite the electrons of a fluorochrome. For example, fluorescein will fluoresce when hit by light with any wavelength between 450 nm and 520 nm. However, the closer the excitation wavelength is to 495 nm, the more fluorescence will be produced. This optimal wavelength is called the excitationnpeak. Similarly, the light produced by fluorochromes has a range of wavelengths. The emission of light from fluorescein ranges from 490 nm to 630 nm, and the emission peak is approximately 515 nm. Since the phenomenon of fluorescence was first explained by a British scientist, Sir George Stokes, in 1852, the shift in wavelength from short to long during fluorescence is called “Stokes shift” (Figure 2).
Figure 2. Excitation and emission spectrum of fluorescein. When fluorescein is excited at a wavelength other than its peak excitation (470 nm in this example), the shape of the emission curve (darker green) remains the same, but the relative intensity is reduced. The efficiency of the excitation at 470 nm is 45% of peak excitation.
Some fluorochromes have a small Stokes shift while other fluorescent compounds have large Stokes shifts. For example, the fluorochrome fluorescein can be excited by blue-green light, and its Stokes shift is only about 20 nm, which means that the light emitted is green. This contrasts with another fluorochrome, phycoerythrin, which also can be excited by blue-green light, but has a large Stokes shift. Thus, the light emitted is yellow-orange. In Immunofluorescence, a single wavelength can be used to excite several fluorochromes with different Stokes shifts and thereby produce a variety of fluorescent colors as shown in Figure 3.
Figure 3. Excitation of three spectrally distinct fluorochromes using a single laser line.
The example in Figure 3 shows a single wavelength at 488 nm (blue line) exciting three different fluorochromes identified by their absorption curves on the left of the figure (blue line). Each fluorochrome is excited at a different efficiency and, therefore, the resulting emission will be at different intensities for equivalent fluorochrome concentrations. Knowing the excitation and emission properties of fluorescent compounds makes it possible to select combinations of fluorochromes that will work together. However, for a fluorochrome to be useful in a biological application it must attach to or be contained within a structure of biological significance.
Fluorochromes can be attached to antibodies which will then bind to specific chemical structures on or inside cells. Many other chemical and physical properties of fluorochromes determine when and where these dyes are useful in various biological assays. For example, some of the fluorochromes that bind to DNA , such as Hoechst 33342, can get into living cells, but most DNA -binding fluorochromes cannot get past the cell membrane. Those fluorescent dyes that cannot get past an intact cell membrane, such as propidium iodide (PI), are often used to distinguish live from dead and dying cells.


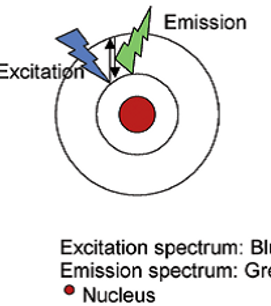
Principle of Fluorescence
The ideal fluorochrome would be a molecule with the following properties:
- A n absorption peak at an excitation wavelength available on the fluorescence detection instrument (large extinction coefficient at the wavelength of excitation)
- Bright fluorescence (high quantum yield)
- A narrow emission spectrum that falls within one of the instrument’s detection bands
- Good photostability
- Fluorescence properties that are not significantly altered by conjugation to an antibody or by the local environment of the sample
How to use immunofluorescence to stain different parts of the cell.